Table of Contents
ToggleBiomass stove engineering design guide
- Designing a biomass stove involves several engineering principles to ensure efficiency, safety, and environmental compliance.
-
Refer Performance Evaluation of Biomass Stove: Efficiency Calculations and Emissions Predictions
-
Controlling Emissions from Biomass Stoves: A Path to Cleaner Cooking
-
Smoke and Dust Minimization Techniques for Briquette and Pellet Stoves
-
- Here’s a comprehensive guide covering key aspects of biomass stove engineering design:
1. Understanding Biomass Fuel
- Types of Biomass: Identify the biomass types (wood, agricultural residues, pellets) and their properties.
- Fuel Characteristics:
- Moisture Content: Affects combustion efficiency; aim for 15-20% moisture.
- Energy Content: Higher Heating Value (HHV) and Lower Heating Value (LHV) are crucial for performance evaluation.
- Bulk Density: Important for determining the size of the fuel storage and combustion chamber.
- Refer the CFD Flow engineering site of the calculation of heating value of biomass coal and wood, biomass waste and pellet
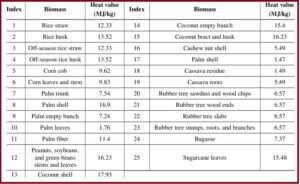
Thermal Design
- Heat Output Calculation:
- Determine the required heat output based on intended applications (e.g., cooking, space heating).
          Q=mf×HHV×ηQÂ
- Combustion Chamber Design:
- Size the combustion chamber to accommodate fuel load and ensure efficient burning.
- Consider factors like firebox volume, depth, and shape for optimal heat distribution.
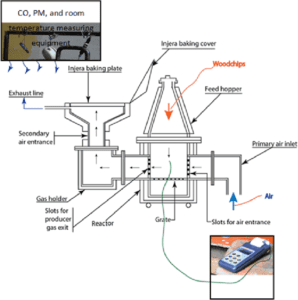
Combustion Efficiency
- Stoichiometric Air Requirement: Calculate the amount of air needed for complete combustion.
- Excess Air Ratio: Use an excess air factor (1.5 to 2) to ensure adequate combustion.
- Efficiency Optimization: Aim for thermal efficiency above 30-40%. Use insulation and reflectors to reduce heat losses.
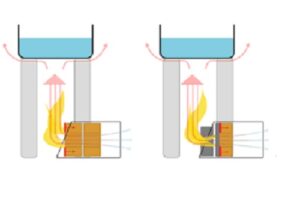
4. Airflow and Draft
- Air Inlet Design: Ensure proper airflow to support combustion; consider adjustable inlets for better control.
- Chimney Design:
- Height and diameter influence draft; use the formula for draft pressure to determine optimal chimney size.
- Ensure the chimney design minimizes back pressure.
Types of biomass pellet burners
Material Selection
- Construction Materials: Choose materials that can withstand high temperatures (e.g., stainless steel, refractory bricks).
- Durability and Safety: Ensure materials are non-toxic and resistant to corrosion and wear.
Emissions Control
- Emission Standards: Research local regulations regarding emissions and design the stove to meet these standards.
- Combustion Chamber Features: Implement baffles or secondary combustion zones to enhance complete combustion and reduce emissions.
- Testing for Emissions: Regularly test for CO, particulate matter (PM), and other emissions.
Safety Considerations
- Stability and Support: Ensure the stove design is stable and can support the weight of the cookware.
- Heat Shielding: Incorporate heat shields or insulation to protect surrounding surfaces from heat damage.
- User Safety Features: Design features such as cool-touch handles and clear visibility of the flame.
User Interface Design
- Ease of Use: Design intuitive controls for air intake and fuel loading.
- Maintenance Accessibility: Ensure that components like ash trays and cleaning ports are easily accessible for maintenance.
Testing and Prototyping
- Prototype Development: Build a prototype for testing performance, emissions, and user experience.
- Field Testing: Conduct tests in real-world conditions to evaluate efficiency and user feedback.
Documentation and Compliance
- User Manuals: Provide clear instructions for use, maintenance, and safety.
- Regulatory Compliance: Ensure design meets relevant safety and environmental standards.
Iterative Design Process
- Feedback Loop: Gather user feedback and performance data to refine and improve the design iteratively.
By following this engineering design guide, you can create a biomass stove that maximizes efficiency, minimizes environmental impact, and provides a safe cooking solution.
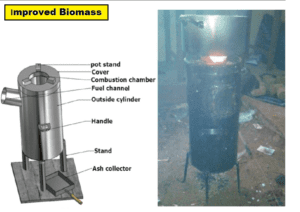
Biomass Stove Combustion Design Calculations
- Designing a biomass stove with optimal combustion involves several key calculations to ensure efficient burning, proper airflow, and minimal emissions.
- Here’s a step-by-step guide for basic combustion design calculations for a biomass stove:
1. Fuel Characterization
- Fuel Type: Identify the biomass fuel (e.g., wood, straw, pellets).
- Moisture Content (MC): Affects the combustion efficiency; should be accounted for in calculations. Typically, 15-20% moisture is common for biomass.
- Energy Content (Higher Heating Value, HHV): Measure the energy content in MJ/kg. For wood, HHV is around 18-20 MJ/kg.
- Fuel Density: Know the bulk density (kg/m³) of the biomass.
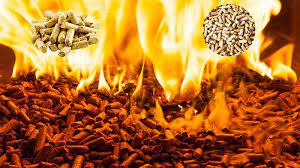
Example:
- HHV of wood = 19 MJ/kg
- MC = 15%
- Bulk density = 500 kg/m³
Combustion Air Requirement
To achieve complete combustion, biomass requires a specific amount of air, mainly oxygen.
- Stoichiometric Air Requirement: calculated the Stoichiometric air requirement based Carbon, hydrogen, and oxygen fractions in the biomass.
- Excess Air Factor (EA):
- Typically, 1.5 to 2 times the stoichiometric air is used for practical combustion.
- Total air required: Air required=Stoichiometric air × Excess air factoÂ
Example:
- C=50%, H=6%, O=40Â
- Stoichiometric air requirement for 1 kg of fuel ≈ 5 kg air/kg fuel.
- With an excess air factor of 1.5, air required ≈ 7.5 kg air/kg fuel.
Combustion Efficiency
- Efficiency is affected by fuel moisture and heat losses. The thermal efficiency can be estimated as:
           η= Useful heat Heat Duty/ Heat Input​​where:
- useful​ heat is the heat transferred to the pot or surroundings.
- Heat input​ is the total heat from biomass combustion.
Typical efficiencies for well-designed stoves range from 25% to 40%.
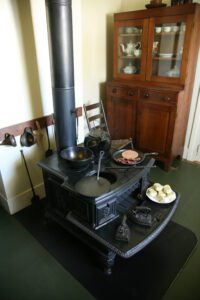
Chimney and Draft Design
- Chimney Height (H):
- To create sufficient draft, chimney height should be optimized using:
- Draft pressure(ΔP)=0.0036×H×(1/To−1/Ta)
- HHH = height of the chimney (m),
- To​ = temperature inside chimney (K),
- Ta= ambient temperature (K).
Suction volume of chimney in kitchen
- To create sufficient draft, chimney height should be optimized using:
Heat Transfer
- Heat Transfer Rate (Q): Q=mf×HHV×ηQÂ
- mf is the mass flow rate of fuel (kg/s).
- HHVÂ is the higher heating value of biomass (MJ/kg).
- η is the thermal efficiency.
Stove Sizing
- Firebox Volume (V): V=mf × burn time/bulk density
- Ensure the firebox size can handle enough fuel for the intended cooking duration.
- You can refer the post for various Biomass stoves used in India and other countries
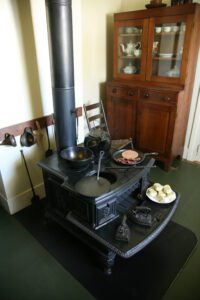
Emissions Calculation
To estimate emissions like COâ‚‚ or CO:
- CO₂ Production: CO₂ emissions=44×Cf12×mf=1244×Cf​​×mf​
For carbon monoxide (CO), you would typically measure the incomplete combustion products based on real-time stove performance.
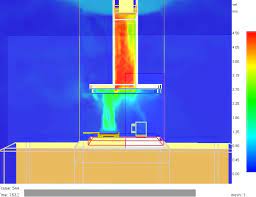
Scope of CFD for biomass stove design
- The scope of Computational Fluid Dynamics (CFD) in biomass stove design is extensive and can significantly enhance the understanding and optimization of the combustion process.
- Here are key areas where CFD can be applied:
Combustion Modeling
- Combustion Efficiency: CFD can simulate the combustion process to evaluate how different designs affect efficiency. This includes analyzing the impact of fuel type, moisture content, and combustion chamber geometry.
- Chemical Reaction Dynamics: Model the chemical reactions occurring during biomass combustion, including the formation of pollutants (e.g., CO, NOx, particulate matter).
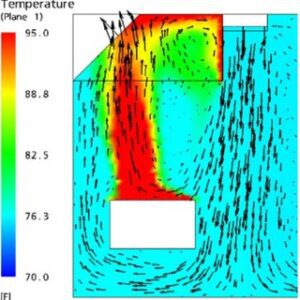
Flow Dynamics
- Airflow Patterns: Analyze airflow within the stove, including how air enters the combustion chamber and interacts with the fuel. This helps in optimizing air inlet designs for better combustion.
- Draft Calculation: Simulate the effects of chimney height and diameter on draft, ensuring sufficient airflow for complete combustion.
Temperature Distribution
- Heat Transfer Analysis: CFD can provide insights into temperature distribution within the stove, helping to identify hot and cold spots. This information is crucial for optimizing heat transfer to the cooking surface or living space.
- Thermal Efficiency: Evaluate the overall thermal efficiency by modeling heat losses through stove materials and external surfaces.
Emission Analysis
- Pollutant Formation: Use CFD to predict the formation of emissions during combustion and assess design changes that can reduce pollutants.
- Emission Dispersion: Simulate how emissions disperse in the surrounding environment, aiding in compliance with environmental regulations.
Design Optimization
- Geometry Modification: Test various stove designs and geometries using CFD to determine the most efficient and effective configuration before physical prototyping.
- Performance Testing: Assess how modifications to components (e.g., baffles, heat exchangers) impact performance, helping to fine-tune designs.
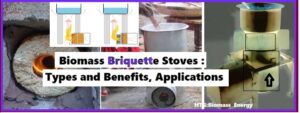
Multi-Phase Flow Simulation
- Fuel Behavior: Model the behavior of solid fuel particles during combustion, including their ignition, combustion, and ash production.
- Moisture Impact: Analyze the effects of varying moisture content in biomass on combustion performance and emissions.
User Interaction and Usability
- User-Centric Design: Simulate real-world usage scenarios to evaluate how design changes impact usability and user experience (e.g., ease of loading fuel, ash removal).
Validation and Testing
- Experimental Validation: Use CFD simulations to complement experimental data, helping to validate design assumptions and performance predictions.
- Predictive Modeling: Create predictive models to forecast performance based on different operating conditions and user behaviors.
Sustainability Assessment
- Life Cycle Analysis (LCA): Incorporate CFD findings into broader sustainability assessments to evaluate the environmental impact of different stove designs throughout their lifecycle.
Integration with Other Technologies
- Hybrid Systems: Model how biomass stoves can be integrated with other renewable technologies (e.g., solar thermal systems) for improved efficiency and performance.
Conclusion
- Utilizing CFD in biomass stove design provides valuable insights that enhance efficiency, reduce emissions, and improve overall performance.
- By simulating and analyzing various parameters, designers can make informed decisions that lead to better biomass stove solutions tailored to user needs and environmental standards.
Reference
- Handbook for Biomass Cookstove in Research, Design, and Development
- Pol et, Design, Fabrication and Testing of a Forced Draft Biomass Cook Stove, IJARSCT (2021)